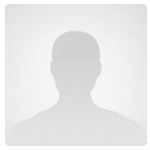
Serena Carra
e-mail: s.carra AT med.umcg.nl
affiliation: Università di Modena-Reggio Emilia
research area(s): Cell Biology, Molecular Biology
Course:
Molecular and Regenerative Medicine
University/Istitution: Università di Modena-Reggio Emilia
University/Istitution: Università di Modena-Reggio Emilia
July 2011- Researcher/Assistant Professor, Iniversita'degli studi di Modena e Reggio Emilia
June 2009-July 2011 Assistant Professor, University Medical Center Groningen
June-2007-May 2009 Post-doctoral fellow, University Medical Center Groningen
Feb.2004-April 2007 Post-doctoral fellow, under the supervision of Prof. Jacques Landry, Centre de recherche L'Hôtel-Dieu de Québec, Laval University, Québec, Canada.
2004 Ph.D. in Neurobiology, University of Catania, Italy.
Oct. 1999 Specialization in Pharmaceutical Research Methods, University of Modena.
Oct. 1998 M.Sc. in Chemistry and Pharmaceutical Sciences, University of Modena, Italy, graduated Oct., 14th 1998, honours with distinctions.
June 2009-July 2011 Assistant Professor, University Medical Center Groningen
June-2007-May 2009 Post-doctoral fellow, University Medical Center Groningen
Feb.2004-April 2007 Post-doctoral fellow, under the supervision of Prof. Jacques Landry, Centre de recherche L'Hôtel-Dieu de Québec, Laval University, Québec, Canada.
2004 Ph.D. in Neurobiology, University of Catania, Italy.
Oct. 1999 Specialization in Pharmaceutical Research Methods, University of Modena.
Oct. 1998 M.Sc. in Chemistry and Pharmaceutical Sciences, University of Modena, Italy, graduated Oct., 14th 1998, honours with distinctions.
Background
Accumulation of aggregated proteins is a pathological hallmark of neurodegenerative and muscular disorders, including the polyglutamine disorders (e.g. Huntington’s Disease and Spinocerebellar Ataxias) and the protein aggregate myopathies (e.g. desmin-related myopathy, muscular dystrophy). Molecular chaperones, which recognize and bind misfolded proteins, prevent their aggregation and facilitate their degradation, thus exerting neuroprotective functions.
Our research project focuses on the chaperone HspB8, which forms a stable complex with the co-chaperone Bag3. Interestingly, mutations in HspB8 and Bag3 cause dominant hereditary peripheral neuropathy and/or muscular dystrophy. We previously demonstrated that overexpression of the HspB8-Bag3 complex prevents the aggregation of mutated huntingtin and facilitates its degradation by stimulating autophagy, an essential process for aggregate-prone proteins clearance and neuronal survival. It is thus conceivable that HspB8-Bag3 might play a role in stress-mediated autophagy stimulation and help cells to cope with misfolded-aggregating substrates.
Hypothesis/goals/aims
In particular, our research focuses on:
1) whether and how the modulation of the HspB8-Bag3 function in vivo may protect against polyglutamine disorders. This aspect of the project is addressed using human patient material, cells and Drosophila melanogaster models of polyQ diseases.
2) how the disease-related mutations in HspB8 and Bag3 affect their function in autophagy (haplo-insufficiency leading to loss of function) or/and whether, by aggregating, they cause disease via the gain of toxic functions. These hypotheses are addressed using cells and Drosophila melanogaster as animal model.
Accumulation of aggregated proteins is a pathological hallmark of neurodegenerative and muscular disorders, including the polyglutamine disorders (e.g. Huntington’s Disease and Spinocerebellar Ataxias) and the protein aggregate myopathies (e.g. desmin-related myopathy, muscular dystrophy). Molecular chaperones, which recognize and bind misfolded proteins, prevent their aggregation and facilitate their degradation, thus exerting neuroprotective functions.
Our research project focuses on the chaperone HspB8, which forms a stable complex with the co-chaperone Bag3. Interestingly, mutations in HspB8 and Bag3 cause dominant hereditary peripheral neuropathy and/or muscular dystrophy. We previously demonstrated that overexpression of the HspB8-Bag3 complex prevents the aggregation of mutated huntingtin and facilitates its degradation by stimulating autophagy, an essential process for aggregate-prone proteins clearance and neuronal survival. It is thus conceivable that HspB8-Bag3 might play a role in stress-mediated autophagy stimulation and help cells to cope with misfolded-aggregating substrates.
Hypothesis/goals/aims
In particular, our research focuses on:
1) whether and how the modulation of the HspB8-Bag3 function in vivo may protect against polyglutamine disorders. This aspect of the project is addressed using human patient material, cells and Drosophila melanogaster models of polyQ diseases.
2) how the disease-related mutations in HspB8 and Bag3 affect their function in autophagy (haplo-insufficiency leading to loss of function) or/and whether, by aggregating, they cause disease via the gain of toxic functions. These hypotheses are addressed using cells and Drosophila melanogaster as animal model.
1. Seidel K, Vinet J, den Dunnen WFA, Brunt ER, Meister M, Boncoraglio A, Zijlstra MP, Boddeke HWGM, Rüb U, Kampinga HH and Carra S. The HSPB8-BAG3 chaperone complex is upregulated in astrocytes in the human brain affected by protein aggregation diseases. Neuropath appl Neurobiol, accepted may 2011.
2. Hishiya A, Salman MN, Carra S, Kampinga HH and Takayama S. BAG3 directly interacts with mutated alphaB-crystallin to suppress its aggregation and toxicity. PLOS ONE, accepted for publication on January 12th 2011.
3. Carra S, Boncoraglio A, Kanon B, Brunsting JF, Minoia M, Rana A, Vos MJ, Seidel K, Sibon OC, Kampinga HH. Identification of the Drosophila ortholog of HSPB8: implication of HSPB8 loss of function in protein folding diseases. J Biol Chem. 2010. Nov 26;285(48):37811-22.
4. Vos MJ, Zijlstra MP, Carra S, Sibon OC, Kampinga HH. Small heat shock proteins, protein degradation and protein aggregation diseases. Autophagy. 2011. 7(1). 101-103
5. Vos MJ, Zijlstra MP, Kanon B, van Waarde-Verhagen MA, Brunt ER, Oosterveld-Hut HM, Carra S, Sibon OC, Kampinga HH. HSPB7 is the most potent polyQ aggregation suppressor within the HSPB family of molecular chaperones. Hum Mol Genet. 2010. 19(23):4677-93.
6. Crippa V, Carra S, Rusmini P, Sau D, Bolzoni E, Bendotti C, De Biasi S, Poletti A. A role of small heat shock protein B8 (HSPB8) in the autophagic removal of misfolded proteins responsible for neurodegenerative diseases. Autophagy. 2010. 6(7):958-60.
7. Crippa V, Sau D, Rusmini P, Boncoraglio A, Onesto E, Bolzoni E, Galbiati M, Fontana E, Marino M, Carra S, Bendotti C, De Biasi S, Poletti A. The small heat shock protein B8 (HSPB8) promotes autophagic removal of misfolded proteins involved in amyotrophic lateral sclerosis (ALS). Hum Mol Genet. 2010. 19(17):3440-56.
8. Sun X, Fontaine JM, Hoppe AD, Carra S, DeGuzman C, Martin JL, Simon S, Vicart P, Welsh MJ, Landry J, Benndorf R. Abnormal interaction of motor neuropathy-associated mutant HspB8 (Hsp22) forms with the RNA helicase Ddx20 (gemin3). Cell Stress & Chaperones, 2010. 15(5) 567-582
9. Fuchs M, Poirier DJ, Seguin S, Lambert H, Carra S, Charrette SJ, Landry J. Identification of the key structural motifs involved in HspB8/HspB6-Bag3 interaction. Biochem J. 2009. 425(1):245-55.
10. Carra S and Kampinga HH. Cytoprotective function of small stress proteins in conformational disorders. In Review Book: Small Stress Proteins and Human Diseases. Chapter 1.1, 2010, Nova Publishers.
11. Carra S, The stress-inducible HspB8-Bag3 complex induces the eIF2alpha kinase pathway: Implications for protein quality control and viral factory degradation? Autophagy. 2009. 5(3):428-9.
12. Carra S, Brunsting JF, Lambert H, Landry J, Kampinga HH. HspB8 participates in protein quality control by a non chaperone-like mechanism that requires eIF2alpha phosphorylation. J Biol Chem. 2008. 284(9): 5523-32.
13. Hageman J, Vos MJ,Carra S, Kampinga HH. Structural and functional homologies and diversities between members of the human HspH (Hsp110), HspA (Hsp70), DnaJ (Hsp40) and HspB (Hsp27) families. Biochemistry, 2008. 47(27):7001-11. Review.
14. Carra S, Seguin SJ and Landry J. HspB8 and Bag3: a new chaperone complex targeting misfolded proteins to macroautophagy. Autophagy. 2008. 4(2):237-9.
15. Carra S, Seguin SJ, Lambert H and Landry J. HspB8 chaperone activity toward poly(Q)-containing proteins depends on its association with Bag3, a stimulator of macroautophagy. J Biol Chem. 2008. 283(3):1437-44.
16. Carra S & Landry J. Role of HspB1 and HspB8 in hereditary peripheral neuropathies: beyond the chaperone function. In Review Book: Heat shock proteins and the brain: implications for neurodegenerative diseases and neuroprotection; Chapter 7, 139-155, 2008, Editors: Asea AAA, Brown IR.
17. Carra S & Landry J. Small Heat Shock Proteins in Neurodegenerative Diseases. In Review Book: Heat Shock Proteins in Biology and Medicine; Section VI, Chapter 18, 331-352, 2006, Editors: Jurgen Radons and Gabriele Multhoff.
18. Carra S, Sivilotti M, Chavez-Zobel AT, Lambert H, Landry J. HspB8, a small heat shock protein mutated in human neuromuscular disorders, has in vivo chaperone activity in cultured cells. Hum. Mol. Genetics, 2005. 14(12):1659-69.
2. Hishiya A, Salman MN, Carra S, Kampinga HH and Takayama S. BAG3 directly interacts with mutated alphaB-crystallin to suppress its aggregation and toxicity. PLOS ONE, accepted for publication on January 12th 2011.
3. Carra S, Boncoraglio A, Kanon B, Brunsting JF, Minoia M, Rana A, Vos MJ, Seidel K, Sibon OC, Kampinga HH. Identification of the Drosophila ortholog of HSPB8: implication of HSPB8 loss of function in protein folding diseases. J Biol Chem. 2010. Nov 26;285(48):37811-22.
4. Vos MJ, Zijlstra MP, Carra S, Sibon OC, Kampinga HH. Small heat shock proteins, protein degradation and protein aggregation diseases. Autophagy. 2011. 7(1). 101-103
5. Vos MJ, Zijlstra MP, Kanon B, van Waarde-Verhagen MA, Brunt ER, Oosterveld-Hut HM, Carra S, Sibon OC, Kampinga HH. HSPB7 is the most potent polyQ aggregation suppressor within the HSPB family of molecular chaperones. Hum Mol Genet. 2010. 19(23):4677-93.
6. Crippa V, Carra S, Rusmini P, Sau D, Bolzoni E, Bendotti C, De Biasi S, Poletti A. A role of small heat shock protein B8 (HSPB8) in the autophagic removal of misfolded proteins responsible for neurodegenerative diseases. Autophagy. 2010. 6(7):958-60.
7. Crippa V, Sau D, Rusmini P, Boncoraglio A, Onesto E, Bolzoni E, Galbiati M, Fontana E, Marino M, Carra S, Bendotti C, De Biasi S, Poletti A. The small heat shock protein B8 (HSPB8) promotes autophagic removal of misfolded proteins involved in amyotrophic lateral sclerosis (ALS). Hum Mol Genet. 2010. 19(17):3440-56.
8. Sun X, Fontaine JM, Hoppe AD, Carra S, DeGuzman C, Martin JL, Simon S, Vicart P, Welsh MJ, Landry J, Benndorf R. Abnormal interaction of motor neuropathy-associated mutant HspB8 (Hsp22) forms with the RNA helicase Ddx20 (gemin3). Cell Stress & Chaperones, 2010. 15(5) 567-582
9. Fuchs M, Poirier DJ, Seguin S, Lambert H, Carra S, Charrette SJ, Landry J. Identification of the key structural motifs involved in HspB8/HspB6-Bag3 interaction. Biochem J. 2009. 425(1):245-55.
10. Carra S and Kampinga HH. Cytoprotective function of small stress proteins in conformational disorders. In Review Book: Small Stress Proteins and Human Diseases. Chapter 1.1, 2010, Nova Publishers.
11. Carra S, The stress-inducible HspB8-Bag3 complex induces the eIF2alpha kinase pathway: Implications for protein quality control and viral factory degradation? Autophagy. 2009. 5(3):428-9.
12. Carra S, Brunsting JF, Lambert H, Landry J, Kampinga HH. HspB8 participates in protein quality control by a non chaperone-like mechanism that requires eIF2alpha phosphorylation. J Biol Chem. 2008. 284(9): 5523-32.
13. Hageman J, Vos MJ,Carra S, Kampinga HH. Structural and functional homologies and diversities between members of the human HspH (Hsp110), HspA (Hsp70), DnaJ (Hsp40) and HspB (Hsp27) families. Biochemistry, 2008. 47(27):7001-11. Review.
14. Carra S, Seguin SJ and Landry J. HspB8 and Bag3: a new chaperone complex targeting misfolded proteins to macroautophagy. Autophagy. 2008. 4(2):237-9.
15. Carra S, Seguin SJ, Lambert H and Landry J. HspB8 chaperone activity toward poly(Q)-containing proteins depends on its association with Bag3, a stimulator of macroautophagy. J Biol Chem. 2008. 283(3):1437-44.
16. Carra S & Landry J. Role of HspB1 and HspB8 in hereditary peripheral neuropathies: beyond the chaperone function. In Review Book: Heat shock proteins and the brain: implications for neurodegenerative diseases and neuroprotection; Chapter 7, 139-155, 2008, Editors: Asea AAA, Brown IR.
17. Carra S & Landry J. Small Heat Shock Proteins in Neurodegenerative Diseases. In Review Book: Heat Shock Proteins in Biology and Medicine; Section VI, Chapter 18, 331-352, 2006, Editors: Jurgen Radons and Gabriele Multhoff.
18. Carra S, Sivilotti M, Chavez-Zobel AT, Lambert H, Landry J. HspB8, a small heat shock protein mutated in human neuromuscular disorders, has in vivo chaperone activity in cultured cells. Hum. Mol. Genetics, 2005. 14(12):1659-69.
Project Title:
Project Title:
Chaperonopathies: how can mutations in small HSP cause disease?
HSPB8 and BAG3 may interact with and modulate the function of other members of the HSPB family that are also highly expressed in skeletal cells and can interact with sarcomeric proteins. This is suggested by the observation that mutated HSPB8 strongly interacts with and sequesters HSPB1 (as compared to wild-type HSPB8), which plays a role in neuron survival. Also, we recently found that mutated R120G HSPB5, associated with desmin-related myopathy, preferentially binds to BAG3. Thus, a dynamic exchange of HSPB8 and other HSPB subunits may occur and other HSPB-BAG3 complex may exist, especially in the skeletal muscle cells, where they may exert specific functions to maintain proteostasis. A perturbation/imbalance in the interactions between HSPB8 and other HSPB proteins and between BAG3 and other HSPB proteins may also participate in the progression of neuro/muscular disorders.
The first part of this project aim at dissecting the interplay between several HSPBs involved in /associated with motor neuropathy or muscular dystrophy and BAG3 and the consequences of such interplay for disease progression.
Two mutations in HSPB8 (K141E and K141N) have been associated with dominant hereditary peripheral neuropathy, whereas two mutations in BAG3 (P209L and R258W) have been associated with muscular dystrophy/myofibrillar myopathy [19, 20]. Intriguingly, both mutated HSPB8 and BAG3 are found in aggregates when overexpressed in mammalian cells. Aggregation of mutated proteins is a common factor of many neuronal and muscular disorders (e.g. it has been documented in PMP22, peripheral myelin protein 22-associated Charcot-Marie-Tooth Disease type 1A and mutated dysferlin-associated muscular dystrophy). Like for mutated polyglutamine proteins, autophagy has been shown to participate also in the clearance of mutated PMP22 and dysferlin aggregates. In principle, two possibilities exist by which HSPB8 and/or BAG3 mutated forms may cause disease: a) mutations of HSPB8 and BAG3 affect their function in PQC via a loss of function mechanism; b) the mutations of HSPB8 and BAG3 cause the proteins to be instable and aggregation-prone, leading to a toxic gain of function. Of course, a combination of these two mechanisms may also apply. With regards to this them, however, we have already shown that both mutated forms of HSPB8 are characterized by a loss of their function in PQC and autophagy, both in cells and in vivo (Dm). Similar studies have already been initiated for transgenic flies expressing wild-type and mutated BAG3, that we have recently generated.
Despite that we have found indications for a loss of function of the mutated forms of HSPB8, our data yet cannot exclude that a gain of toxic function plays no role in disease. So far, our data were limited mainly to cell line work and in vivo targeting the expression of the mutated forms of HSPB8 to the fly eye; it could very well be that the K141E and K141N HSPB8 may gain toxic functions only in the specific tissues that are affected in disease (e.g. motor neurons and skeletal muscle). We will therefore continue the characterization of our transgenic flies expressing wild-type and mutated HSPB8 or BAG3 selectively targeting their expression to motor neurons and skeletal muscle cells. As readouts, we will -amongst others- use: 1) fly locomotor behaviour; 2) presence/accumulation of autophagic vacuoles; 3) presence of polyubiquitinated and aggregated proteins; 4) integrity of Z-disk and other cytoskeletal elements at the ultrastructural level.
The first part of this project aim at dissecting the interplay between several HSPBs involved in /associated with motor neuropathy or muscular dystrophy and BAG3 and the consequences of such interplay for disease progression.
Two mutations in HSPB8 (K141E and K141N) have been associated with dominant hereditary peripheral neuropathy, whereas two mutations in BAG3 (P209L and R258W) have been associated with muscular dystrophy/myofibrillar myopathy [19, 20]. Intriguingly, both mutated HSPB8 and BAG3 are found in aggregates when overexpressed in mammalian cells. Aggregation of mutated proteins is a common factor of many neuronal and muscular disorders (e.g. it has been documented in PMP22, peripheral myelin protein 22-associated Charcot-Marie-Tooth Disease type 1A and mutated dysferlin-associated muscular dystrophy). Like for mutated polyglutamine proteins, autophagy has been shown to participate also in the clearance of mutated PMP22 and dysferlin aggregates. In principle, two possibilities exist by which HSPB8 and/or BAG3 mutated forms may cause disease: a) mutations of HSPB8 and BAG3 affect their function in PQC via a loss of function mechanism; b) the mutations of HSPB8 and BAG3 cause the proteins to be instable and aggregation-prone, leading to a toxic gain of function. Of course, a combination of these two mechanisms may also apply. With regards to this them, however, we have already shown that both mutated forms of HSPB8 are characterized by a loss of their function in PQC and autophagy, both in cells and in vivo (Dm). Similar studies have already been initiated for transgenic flies expressing wild-type and mutated BAG3, that we have recently generated.
Despite that we have found indications for a loss of function of the mutated forms of HSPB8, our data yet cannot exclude that a gain of toxic function plays no role in disease. So far, our data were limited mainly to cell line work and in vivo targeting the expression of the mutated forms of HSPB8 to the fly eye; it could very well be that the K141E and K141N HSPB8 may gain toxic functions only in the specific tissues that are affected in disease (e.g. motor neurons and skeletal muscle). We will therefore continue the characterization of our transgenic flies expressing wild-type and mutated HSPB8 or BAG3 selectively targeting their expression to motor neurons and skeletal muscle cells. As readouts, we will -amongst others- use: 1) fly locomotor behaviour; 2) presence/accumulation of autophagic vacuoles; 3) presence of polyubiquitinated and aggregated proteins; 4) integrity of Z-disk and other cytoskeletal elements at the ultrastructural level.
Project Title:
Further dissecting the role of the HSPB8-BAG3 complex in the modulation of protein homeostasis and autophagy: implications in disease
HSPB8 is a member of the human family of small heat shock proteins (sHSPs; HSPB1-HSPB10). HSPB8 forms a stable complex with the HSP70/HSC70 co-chaperone BAG3. We demonstrated that overexpression of the HSPB8-BAG3 complex prevents the aggregation of mutated huntingtin (associated with Huntington disease), mutated SOD1 and TDP-43 (associated with Amyotrophic Lateral Sclerosis; in collaboration with Prof. A. Poletti, University of Milan) and facilitates their degradation by stimulating autophagy. Autophagy is a degradation process that involves the engulfment and digestion of portions of the cytoplasm in double-membraned vesicles (autophagic vacuoles). Autophagy plays a major role in the degradation of microaggregated proteins (e.g. mutated polyQ proteins) and is essential for neuronal survival. Thus, by modulating protein quality control and stress-mediated autophagy, HSPB8-BAG3 may help the cells to cope with misfolded proteins. From our previous work, we know that HSPB8-BAG3 induces autophagy via the translation initiation factor eIF2-alpha pathway. Besides stimulating autophagy (which facilitates the clearance of microaggregated proteins), the induction of eIF2-alpha phosphorylation causes the inhibition of protein synthesis. Protein synthesis inhibition decreases the load of mutated proteins for the cells to an amount manageable by the molecular chaperones, thus also playing a critical role for the maintenance of protein homeostasis (as recently demonstrated by Tsaytler P. et al.).
This project aims at further investigating, at the molecular level, the role of the HSPB8-BAG3 complex in the maintenance of protein homeostasis. We will dissect the precise mode of action by which BAG3 seems to orchestrate the cellular responses under different conditions of proteotoxic stress and in different models for protein aggregation diseases.
The implications of these cellular findings in proteastasis and disease will be next addressed using Drosophila melanogaster transgenic lines expressing mutated polyglutamine proteins, mutated TDP-43 (in collaboration with Dr. L. Luheshi, University of Cambridge and with Prof. H.H. Kampinga, University of Groningen) and human HSPB8 or BAG3 that we have generated.
This project aims at further investigating, at the molecular level, the role of the HSPB8-BAG3 complex in the maintenance of protein homeostasis. We will dissect the precise mode of action by which BAG3 seems to orchestrate the cellular responses under different conditions of proteotoxic stress and in different models for protein aggregation diseases.
The implications of these cellular findings in proteastasis and disease will be next addressed using Drosophila melanogaster transgenic lines expressing mutated polyglutamine proteins, mutated TDP-43 (in collaboration with Dr. L. Luheshi, University of Cambridge and with Prof. H.H. Kampinga, University of Groningen) and human HSPB8 or BAG3 that we have generated.