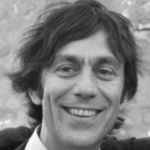
Marco Muzi-Falconi
e-mail: marco.muzifalconi AT unimi.it
affiliation: Dept of Biomolecular Science and Biotechnology
research area(s): Molecular Biology, Genetics And Genomics
Course:
Biomolecular Sciences
University/Istitution: Università di Milano
University/Istitution: Università di Milano
Marco Muzi-Falconi was born in Milano, Italy, in 1965. He received the degree in Biological Sciences (summa cum laude) and the Ph.D. degree in Cellular and Molecular Biology from the Università degli Studi di Milano, Italy, in 1989 and 1993, respectively. From 1993 to 1997 Dr. Muzi-Falconi has been a Post-Doc at the Department of Molecular Biology and Genetics, Johns Hopkins University, School of Medicine, Baltimore USA. Since 1998 Dr. Muzi-Falconi has been leading a research group at the Università degli Studi di Milano, where he is now Associate Professor of Molecular Biology. Dr. Muzi-Falconi has worked for several years on the mechanisms controlling initiation of eukaryotic DNA replication during the cell cycle. Later on, using budding yeast and culture cells as model systems, he started investigating the cell cycle chekpoints, genetically controlled mechanisms that coordinate DNA repair, DNA replication, recombination with cell cycle progression. He also has an interest in mechanisms controlling mitosis and mitochondrial function.
REGULATION OF INITIATION OF DNA REPLICATION
During his stay at Johns Hopkins University, M. Muzi-Falconi investigated the players and processes involved in regulating the initiation of DNA replication at an origin, with a particular emphasis toward the identification of the factors that oversee the crucial control that allows each origin to be fired once and only once per cell cycle. These studies led to the identification of fission yeast Cdc18 as a key regulator of origin firing and allowed the determination of a mechanism, based on Cdc18 phosphorylation, restricting origin firing to only once per cell cycle. Finally, this work allowed the identification, cloning and characterization of the fission yeast homolog of the large subunit of the Origin Recognition Complex (ORC). This was the first ORC-like gene identified in an organism different from S.cerevisiae, proving that, albeit no evolutionary conservation can be detected in replication origins, the factors acting in trans are, on the other hand, well conserved.
MOLECULAR MECHANISMS OF DNA DAMAGE CHECKPOINTS
Upon the set-up of his own group, M. Muzi-Falconi devoted most of his research to the characterization of the mechanisms that underlie the cellular response to DNA damage. This work has seen the use of budding yeast as a model system, where the molecular details are more easily determined. Next, once these processes have been identified and understood in yeast, experiments are performed in human cells to probe the same pathways and possibly extend the findings obtained in yeast. This has proved to be a successful approach.
The preservation of the integrity of the eukaryotic genome is supported by repair mechanisms and require surveillance mechanisms, called checkpoints, that integrate cell cycle progression, DNA repair, replication and recombination. The DNA damage checkpoint is a signaling pathway, activated in response to genomic insults, that results in a cell cycle delay, slow down of DNA replication, activation of repair mechanisms, modification of the cellular transcriptional program.
The work carried out in M. Muzi-Falconi’s group has led to the definition of the molecular details of the phosphorylation-based signal transduction cascade that sits at the core of the checkpoint response. Critical modification of histones were identified and the crosstalk between DNA damage checkpoint and chromatin structure/modification was studied. Moreover, this work allowed the determination of the actual mechanism that allows cells to detect UV lesions and process them to properly activate the apical checkpoint kinase. Furthermore, the involvement of Exo1 nuclease in the checkpoint response and it’s regulation by checkpoint factors and chromatin structure was characterized.
MECHANISMS PRESERVING GENOME INTEGRITY
Several other processes also participate to the maintenance of genome stability. M. Muzi Falconi’s lab has been investigating the cellular response to replication stress, the regulation of post-replication repair (PRR) and the mechanism controlling the proper orientation of the mitotic spindle.
Replication stress is generated when the replication fork encounters problems, these may be, for example, DNA lesions in the template strand, exhaustion of dNTP pools, accumulation of topological constraints. Under these conditions a checkpoint response is activated, which targets the replication factors and likely coordinates the PRR pathways. The work carried out in M.Muzi-Falconi lab has revealed the connections between the 9-1-1 checkpoint clamp and the Dpb11 replication factor in response to stress, suggesting that the former senses problems affecting the lagging strand, while the latter is specific for stalling on the leading strand. Moreover, the regulation of translesion (TLS) DNA synthesis, a sub-pathway of PRR has always been a mayor interest in the lab and functional connections between the 9-1-1 clamp and TLS polymerases were revealed. More recently, M. Muzi-Falconi has been investigating the role of RNaseH in contributing to the maintenance of genome integrity and its cooperation with PRR pathways.
Another aspect that has been studied in the lab is the role of Haspin kinases in controlling mitosis, with particular emphasis on their role in regulating the correct orientation of the mitotic spindle. Failure of this process, in fact, results in the formation of daughter cells that either contain two nuclei or are anucleated. Haspin is conserved also in human cells, where it contributes to spindle orientation and to the determination of the plane of cell division.
During his stay at Johns Hopkins University, M. Muzi-Falconi investigated the players and processes involved in regulating the initiation of DNA replication at an origin, with a particular emphasis toward the identification of the factors that oversee the crucial control that allows each origin to be fired once and only once per cell cycle. These studies led to the identification of fission yeast Cdc18 as a key regulator of origin firing and allowed the determination of a mechanism, based on Cdc18 phosphorylation, restricting origin firing to only once per cell cycle. Finally, this work allowed the identification, cloning and characterization of the fission yeast homolog of the large subunit of the Origin Recognition Complex (ORC). This was the first ORC-like gene identified in an organism different from S.cerevisiae, proving that, albeit no evolutionary conservation can be detected in replication origins, the factors acting in trans are, on the other hand, well conserved.
MOLECULAR MECHANISMS OF DNA DAMAGE CHECKPOINTS
Upon the set-up of his own group, M. Muzi-Falconi devoted most of his research to the characterization of the mechanisms that underlie the cellular response to DNA damage. This work has seen the use of budding yeast as a model system, where the molecular details are more easily determined. Next, once these processes have been identified and understood in yeast, experiments are performed in human cells to probe the same pathways and possibly extend the findings obtained in yeast. This has proved to be a successful approach.
The preservation of the integrity of the eukaryotic genome is supported by repair mechanisms and require surveillance mechanisms, called checkpoints, that integrate cell cycle progression, DNA repair, replication and recombination. The DNA damage checkpoint is a signaling pathway, activated in response to genomic insults, that results in a cell cycle delay, slow down of DNA replication, activation of repair mechanisms, modification of the cellular transcriptional program.
The work carried out in M. Muzi-Falconi’s group has led to the definition of the molecular details of the phosphorylation-based signal transduction cascade that sits at the core of the checkpoint response. Critical modification of histones were identified and the crosstalk between DNA damage checkpoint and chromatin structure/modification was studied. Moreover, this work allowed the determination of the actual mechanism that allows cells to detect UV lesions and process them to properly activate the apical checkpoint kinase. Furthermore, the involvement of Exo1 nuclease in the checkpoint response and it’s regulation by checkpoint factors and chromatin structure was characterized.
MECHANISMS PRESERVING GENOME INTEGRITY
Several other processes also participate to the maintenance of genome stability. M. Muzi Falconi’s lab has been investigating the cellular response to replication stress, the regulation of post-replication repair (PRR) and the mechanism controlling the proper orientation of the mitotic spindle.
Replication stress is generated when the replication fork encounters problems, these may be, for example, DNA lesions in the template strand, exhaustion of dNTP pools, accumulation of topological constraints. Under these conditions a checkpoint response is activated, which targets the replication factors and likely coordinates the PRR pathways. The work carried out in M.Muzi-Falconi lab has revealed the connections between the 9-1-1 checkpoint clamp and the Dpb11 replication factor in response to stress, suggesting that the former senses problems affecting the lagging strand, while the latter is specific for stalling on the leading strand. Moreover, the regulation of translesion (TLS) DNA synthesis, a sub-pathway of PRR has always been a mayor interest in the lab and functional connections between the 9-1-1 clamp and TLS polymerases were revealed. More recently, M. Muzi-Falconi has been investigating the role of RNaseH in contributing to the maintenance of genome integrity and its cooperation with PRR pathways.
Another aspect that has been studied in the lab is the role of Haspin kinases in controlling mitosis, with particular emphasis on their role in regulating the correct orientation of the mitotic spindle. Failure of this process, in fact, results in the formation of daughter cells that either contain two nuclei or are anucleated. Haspin is conserved also in human cells, where it contributes to spindle orientation and to the determination of the plane of cell division.
Sertic S., Cloney R., Lehmann A.R., Marini F., Plevani P. and Muzi-Falconi M. (2011) Human exonuclease 1 connects NER processing with checkpoint activation in response to UV irradiation. Proc Natl Acad Sci USA in press.
Novarina D., Amara F., Lazzaro F., Plevani P. and Muzi-Falconi M. (2011) The cellular response to UV irradiation: a coordinated network involving nucleotide excision repair, post replication repair and DNA damage checkpoints. DNA Repair Epub ahead of print
Puddu F., Piergiovanni G., Plevani P. and Muzi-Falconi M. (2011) Sensing replication stress and Mec1 activation act through two independent pathways involving the 9-1-1 complex and DNA polymerase epsilon. PLoS Genetics 7:e1002022.
Giannattasio M., Tourrière H., Follonier C., Puddu F., Lazzaro F., Pasero P., Lopes M., Plevani P. and Marco Muzi-Falconi (2010) Exo1 competes with repair synthesis, converts NER intermediates to long ssDNA gaps and promotes checkpoint activation. Molecular Cell 40:50-62.
Granata M., Lazzaro F., Novarina D., Panigada D., Puddu F., Grenon M., Kumar R., Lowndes NF., Plevani P. and Marco Muzi-Falconi M. (2010) Dynamics of Rad9 Chromatin Binding and Checkpoint Function Are Mediated by its Dimerization and Are Cell Cycle Regulated by CDK1 Activity. PLoS Genetics 6: pii: e1001047.
Novarina D., Amara F., Lazzaro F., Plevani P. and Muzi-Falconi M. (2011) The cellular response to UV irradiation: a coordinated network involving nucleotide excision repair, post replication repair and DNA damage checkpoints. DNA Repair Epub ahead of print
Puddu F., Piergiovanni G., Plevani P. and Muzi-Falconi M. (2011) Sensing replication stress and Mec1 activation act through two independent pathways involving the 9-1-1 complex and DNA polymerase epsilon. PLoS Genetics 7:e1002022.
Giannattasio M., Tourrière H., Follonier C., Puddu F., Lazzaro F., Pasero P., Lopes M., Plevani P. and Marco Muzi-Falconi (2010) Exo1 competes with repair synthesis, converts NER intermediates to long ssDNA gaps and promotes checkpoint activation. Molecular Cell 40:50-62.
Granata M., Lazzaro F., Novarina D., Panigada D., Puddu F., Grenon M., Kumar R., Lowndes NF., Plevani P. and Marco Muzi-Falconi M. (2010) Dynamics of Rad9 Chromatin Binding and Checkpoint Function Are Mediated by its Dimerization and Are Cell Cycle Regulated by CDK1 Activity. PLoS Genetics 6: pii: e1001047.
Project Title:
Definition of the role of Exo1 in the cellular response to UV lesions in yeast and human cells.
In yeast, NER processing and Exo1 are required to generate ssDNA gaps that recruit checkpoint complexes onto UV-damaged chromosomes . Moreover, yeast Rad14 (XPA homolog) has been shown to interact with the checkpoint protein Ddc1, which also has contacts with Exo1, suggesting that the UV response mechanism may be regulated by controlling protein-protein interactions.
We showed that Exo1 competes with DNA polymerases during the refilling step of NER; when the reaction is slow or blocked, Exo1 captures NER intermediates and generates long ssDNA gaps. This situation can happen when two closely spaced UV lesions are located on both DNA strands (closely opposing lesions). Closely opposing lesions, although being statistically unlikely, are known to exist, and we have suggested that they contribute to DNA damage checkpoint. Accordingly, in non replicating cells TLS polymerases play a crucial role in the refilling step of a portion of the damage sites, which correlates with ubiquitylation of PCNA in G1 arrested UV-irradiated cells. TLS activity may be required at opposing lesions sites to allow refilling in the presence of template lesions, and may thus be tightly correlated to Exo1 function and checkpoint activation. We plan to investigate this aspect by identifying the steps required to achieve ubiquitylation of PCNA in G1 arrested cells, through the use of appropriately mutated yeast strains. We will also define the location of this DNA synthetic activity taking advantage of site specific mutations in DNA polymerase coding genes, that allow the enzyme to bind to the primer:template junction, but prevent dNTP addition, thereby locking the enzyme in place. By ChIP-based approaches, we expect to be able to determine the genomic locations of these trapped enzymes.
A key question is how frequently Exo1 intervenes in lesion processing and how are lesions selected for Exo1 processing. To complement the analysis described above, we wish to define whether the regions where Exo1 acts on UV lesions are randomly distributed in the genome, or they are clustered at some sites that may act as sensor for triggering the checkpoint; there could be a lesion type bias, a strand specific bias (e.g. transcribed vs non-transcribed), a chromatin context bias, etc. We will investigate these possibilities by molecular combing, co-localizing Exo1-dependent BrdU tracks on chromosomes with specific FISH probes, to determine their localization. Conditionally expressing CPDs- or 6,4PP- specific photolyases in UV irradiated cells will allow the selective removal of specific lesions, allowing to test whether Exo1processing is lesion-specific. The effect of lesion removal on checkpoint signaling can be studied by monitoring the kinetics of checkpoint activation by Western blotting with Rad53 Ab or by in situ kinase assays.
Finally, we have developed a first generation algorithm that, given a DNA sequence, predicts the most likely location of CPDs. We are now proposing to include 6,4PP predictions and to adapt the algorithm so that the UV dose (and the number of generated lesions) variable is taken into account, and to expand it to predict in silico the location of closely opposing lesion in a specific sequence. The presence of closely opposing lesions can be verified by conventional methods, and the in silico predictions can be tested initially by ChIP with lesion-specific antibodies, monitoring the enrichment of predicted sequences. If this will give positive results, we will integrate it with ChIP-CHIP analysis to verify genome-wide the predictions of the computational model. This approach would provide us with positional information on closely opposing lesions within the genome, allowing us to perform studies on protein binding, kinetics of factors recruitment, lesion processing etc. using ChIP-based methods.
Given the observations in yeast, we have verified that UV-induced checkpoint requires Exo1 also in human cells. Methods have been developed to UV irradiate confined areas of individual cell nuclei by using filters containing pores of a defined size, and this strategy has been used to demonstrate that hEXO1 is recruited to the sites of lesion upon UV irradiation and that such recruitment requires NER factors and activities. We will investigate the mechanism underlying hEXO1 recruitment at the sites of DNA damage. We will define which NER factors bind hEXO1, through immunoprecipitation studies, and, by GST pulldown analysis we will map the interaction domains, which will provide targets for future site directed mutagenesis. This will be important in order to determine the physiological relevance of these physical contacts. Moreover, the role of PCNA ubiquitylation in hEXO1 recruitment will also be investigated
We showed that Exo1 competes with DNA polymerases during the refilling step of NER; when the reaction is slow or blocked, Exo1 captures NER intermediates and generates long ssDNA gaps. This situation can happen when two closely spaced UV lesions are located on both DNA strands (closely opposing lesions). Closely opposing lesions, although being statistically unlikely, are known to exist, and we have suggested that they contribute to DNA damage checkpoint. Accordingly, in non replicating cells TLS polymerases play a crucial role in the refilling step of a portion of the damage sites, which correlates with ubiquitylation of PCNA in G1 arrested UV-irradiated cells. TLS activity may be required at opposing lesions sites to allow refilling in the presence of template lesions, and may thus be tightly correlated to Exo1 function and checkpoint activation. We plan to investigate this aspect by identifying the steps required to achieve ubiquitylation of PCNA in G1 arrested cells, through the use of appropriately mutated yeast strains. We will also define the location of this DNA synthetic activity taking advantage of site specific mutations in DNA polymerase coding genes, that allow the enzyme to bind to the primer:template junction, but prevent dNTP addition, thereby locking the enzyme in place. By ChIP-based approaches, we expect to be able to determine the genomic locations of these trapped enzymes.
A key question is how frequently Exo1 intervenes in lesion processing and how are lesions selected for Exo1 processing. To complement the analysis described above, we wish to define whether the regions where Exo1 acts on UV lesions are randomly distributed in the genome, or they are clustered at some sites that may act as sensor for triggering the checkpoint; there could be a lesion type bias, a strand specific bias (e.g. transcribed vs non-transcribed), a chromatin context bias, etc. We will investigate these possibilities by molecular combing, co-localizing Exo1-dependent BrdU tracks on chromosomes with specific FISH probes, to determine their localization. Conditionally expressing CPDs- or 6,4PP- specific photolyases in UV irradiated cells will allow the selective removal of specific lesions, allowing to test whether Exo1processing is lesion-specific. The effect of lesion removal on checkpoint signaling can be studied by monitoring the kinetics of checkpoint activation by Western blotting with Rad53 Ab or by in situ kinase assays.
Finally, we have developed a first generation algorithm that, given a DNA sequence, predicts the most likely location of CPDs. We are now proposing to include 6,4PP predictions and to adapt the algorithm so that the UV dose (and the number of generated lesions) variable is taken into account, and to expand it to predict in silico the location of closely opposing lesion in a specific sequence. The presence of closely opposing lesions can be verified by conventional methods, and the in silico predictions can be tested initially by ChIP with lesion-specific antibodies, monitoring the enrichment of predicted sequences. If this will give positive results, we will integrate it with ChIP-CHIP analysis to verify genome-wide the predictions of the computational model. This approach would provide us with positional information on closely opposing lesions within the genome, allowing us to perform studies on protein binding, kinetics of factors recruitment, lesion processing etc. using ChIP-based methods.
Given the observations in yeast, we have verified that UV-induced checkpoint requires Exo1 also in human cells. Methods have been developed to UV irradiate confined areas of individual cell nuclei by using filters containing pores of a defined size, and this strategy has been used to demonstrate that hEXO1 is recruited to the sites of lesion upon UV irradiation and that such recruitment requires NER factors and activities. We will investigate the mechanism underlying hEXO1 recruitment at the sites of DNA damage. We will define which NER factors bind hEXO1, through immunoprecipitation studies, and, by GST pulldown analysis we will map the interaction domains, which will provide targets for future site directed mutagenesis. This will be important in order to determine the physiological relevance of these physical contacts. Moreover, the role of PCNA ubiquitylation in hEXO1 recruitment will also be investigated